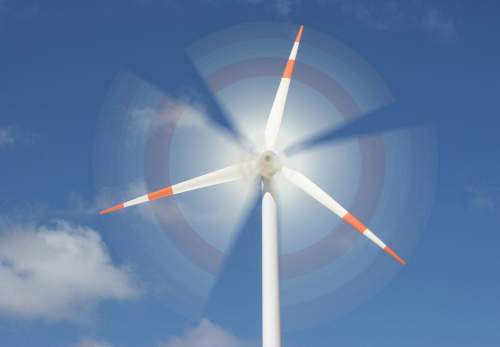
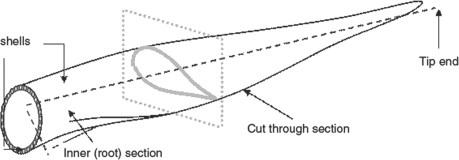
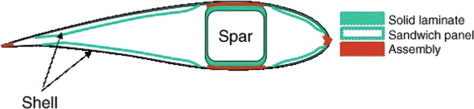





There are two main designs of wind turbine blade. These are outlined on the following page, where we illustrate where the adhesive is applied and explain the different requirements and chemistries of the adhesives used.
Of key importance is the load distribution of forces via the type of blade construction chosen to ensure optimal stress transfer between the adhesive and the composite parts. Generally, a blade consists of two shells which are bonded together with a structural adhesive (Figure 1). The internal structure comprises two different geometries which require two different adhesive chemistries.
A cross-section through a blade gives a better understanding of these two geometries.
One-piece construction In the one-piece blade construction (illustrated in Figure 2), a spar is fixed into the first shell, then the second shell is lifted over onto the spar, thus making the three-component construction (i.e. shell + spar + shell).
The adhesive bonding area is much more important here than in the two-piece construction (Figure 3). The adhesive layer is also subjected to lower stress. Polyurethane (PU) adhesive systems are sufficient to support such stress.
Two-piece construction In this type of blade, two stiffeners or shear webs are bonded into the shells, as shown in Figure 3.
In this construction the adhesive is load bearing, therefore higher mechanical performance is required and consequently epoxy adhesive systems are used.
Adhesive properties
The use of thixotropic materials (in general two-component adhesives) for bonding the blades is essential. The two components – resin and hardener – have to develop ‘non-slump’ properties very quickly after mixing, especially when applied on vertical surfaces in thicknesses of up to 20-30 mm, and maintain the initial shape until gelled or until the next processing step.
This rapid change to a non-slump texture is often achieved by using a thixotropic agent, such as fumed silica, to give a viscous, thixotropic composition. Otherwise, when the resin and the hardener are exposed to high shear conditions, i.e. during their mixing and application steps, the resulting stress can lower viscosity, which can, in turn, lead to distortion of the extruded shape prior to gelling. In some cases, the mixture can even lose its structure and actually run after the stress is removed. This represents one of the limits of current materials based on ‘physical thixotropy.’
The viscosity of the two components is also very high, necessitating the use of high performance pumps for applying the adhesive.
After the installation of turbines, the blades are constantly submitted to vibrations due to wind conditions. Therefore fatigue resistance or toughness are essential adhesive properties to ensure the longevity of the blades. In fact, if the adhesive bond starts to fail, it can not undergo stress anymore and then the internal composite structure of the blade is damaged and fails as well.
Adhesive application
In the wind energy industry, adhesives are commonly applied with two-component metering and mixing equipment (Figure 4). In general, two mix machines are used to apply the adhesive simultaneously to the blade: one starts at the tip end, and the other at the root. The resin and the hardener are mechanically pumped or extruded from their containers, through delivery pipes, to the mixer head where the two components are thoroughly blended and discharged.
The adhesive is applied to the first shell in the fixed part of the mould, and to the second one, at the root end of the blade (Figure 5.1). Then the internal parts (spar or shear webs) are lifted into position (Figure 5.2). The adhesive is applied to the top of the spar or shear webs for the second shell bonding (Figure 5.3). Once all the adhesive application is finished, the second shell is lifted over onto the spar or the shear webs thus completing the construction (Figure 5.4). When the adhesive is applied, ideally it should be maintained in its initial shape until it has gelled or until the next processing step (e.g. closing the mould, Figure 5.5).
Normally, for a PU adhesive, the amount of adhesive used to bond a typical blade (about 42 m) does not exceed 400 kg for an application time which is generally less than 40-45 minutes. In addition, about 15 minutes more are required to complete the construction and the curing time of the adhesive represents about 4 hours at 70-80°C.
During processing, the resin and the hardener are exposed to high shear conditions and such stress can result in a lowering of viscosity, which can lead to distortion of the extruded shape prior to gelling. In some cases, the mixture can even lose its structure and actually run after the stress is removed.
Special adhesives based on the ‘chemical thixotropy’ concept have been developed to allow easy pumping of low viscosity products without slumping when the final blend is applied to a vertical surface after having been exposed to a high shear stress regime. The principle of this patented concept is described below.
Chemical thixotropy concept
This concept relies on specific component combinations: one of the specific components is included in the resin, the other in the hardener, and they do not modify drastically the viscosity of each part. When the resin and hardener are mixed together, the protonation of a high molecular weight cationic polymer by acid entities present on the surface of special fillers occurs and the system develops almost instantaneously a physically cross-linked structure (Figure 7).
Characterisation of the chemical thixotropy
A rheological comparison has been made between three adhesive systems:
- System 1 = Resin + Hardener 1, based on ‘chemical thixotropy’;
- System 2 = Resin + Hardener 2, based on ‘physical thixotropy.’ Hardener 2 has been highly filled with a fumed silica based thixotropic agent so that the System 2 has the same sag resistance on vertical application as the System 1;
- System 3 = Resin + Hardener 3, based on ‘physical thixotropy.’ Hardener 3 has been filled with a fumed silica based thixotropic agent so that the System 3 has roughly the same initial mix viscosity level under low shear rate as with System 1.
It was found that the systems containing the ‘physical thixotropy’ give a lower final viscosity after different shearing steps (low shear to high shear to low shear) even after application of a recovery time whereas the System 1 based on the ‘chemical thixotropy’ presents the same viscosity level before and after shearing.
This shows that the recovery time is different for both technologies. Whereas ‘chemical thixotropy’ is generated on mixing and builds up almost instantaneously after application, in the case of the ‘physical thixotropy’ the structure created by the thixotropic agent is partially destroyed at high shear rate and is not recovered rapidly.
Toughening by nano-particles
There have been many articles in the literature reporting toughening improvement by the incorporation of nano-particles (nanosilicates, carbon nanotubes, nanoclays …) into a matrix resin system. However such systems do not usually show exceptional toughness improvement. In the current adhesive systems, we found a synergetic effect between a special type of organic nano-particles and epoxy systems. This unique combination brings an exceptional toughness improvement.
Toughening property evaluation
Toughening properties of a new tough epoxy adhesive based on the above nanotechnology were measured by three different test methods (see below) for comparison with those of a standard epoxy system.
Bend Notch test The Bend Notch test is a method where the result reveals the outstanding toughness of the system. While other common properties of the new adhesive are comparable to those of the standard system, the fracture energy (G1c) value for our new adhesive is four times that of the standard one.
Fatigue crack measurements The adhesive material within a representative composite structure is tested with fatigue measurements by means of a servo-hydraulic machine. The aim of these tests is to simulate the working of blades subjected to wind in very severe operating conditions, and thus to predict the ultimate behaviour of the adhesive material inside: damage with cracks initiation.
It has been shown that the toughened system is more resistant to damage initiation than the standard material with increasing numbers of cycles. For instance, after one million cycles in severe conditions, the standard adhesive material shows at least twice as many cracks as those in the toughened system.
Dynamic tensile mechanical spectrometry This original procedure, developed by Huntsman, is performed on the different adhesive materials by using a viscoanalyser. The influence of the dynamic strain (e dyn) on the elastic or real part (E') of the complex Young's modulus (E*) is precisely analysed for a constant frequency at room temperature. In fact, the dynamic strain (e dyn) increases continuously in order to promote damage by production of microcracks within the adhesive materials and then, their failure.
Then a decrease of the elastic part (E') can be seen as function of the dynamic strain for the different systems investigated. Nevertheless, this decrease is more pronounced for the standard sample whereas this evolution remains more progressive and gentle in the toughened sample.
This result means that this latter is more able to absorb the deformation applied. In addition, a weak resistance to damage initiation or crack initiation is highlighted for the standard system in contrast with the excellent resistance for the toughened system.
Finally, it is shown that the tough-ened additives increase the fatigue resistance of the toughened adhesive by a factor of 400%.
Other markets
We have demonstrated that chemical thixotropy and nanotechnology are a breakthrough technique in the application of bonding wind turbine blades. The concept of chemical thixotropy to produce adhesives with controlled flow and organic nanotechnology to enhance the toughness have been successfully applied in developing adhesives for use in bonding wind turbine blades.
Applications in other markets are under investigation.