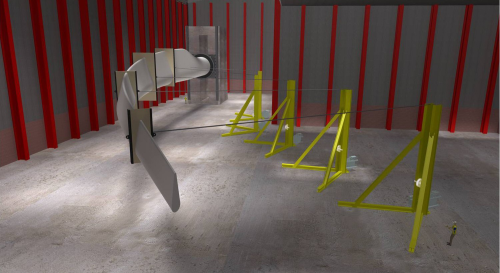
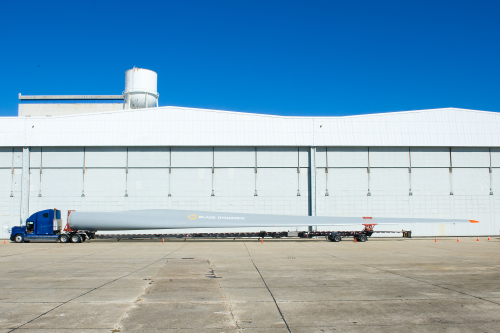
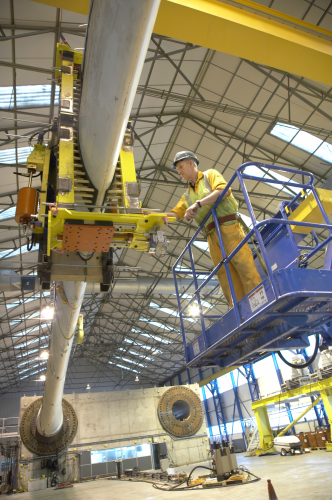
The National Renewable Energy Centre (Narec) is the UK's national research centre for accelerating grid integration of renewable energy. Its wind turbine blade test facility can test blades up to 50 m in length and has been operational since 2005. A 100 m blade testing facility is currently under construction and is expected to be commissioned in spring 2012.
In the United States, the National Renewable Energy Laboratory's (NREL) Structural Testing Laboratory at the National Wind Technology Center in Colorado has two blade stands. Tests include ultimate static strength, fatigue, vibration and non-destructive tests for blades up to 50 m long. The facility has tested blades for well-known wind turbine manufacturers such as GE, Clipper, TPI and Mitsubishi in the past.
A Wind Technology Testing Center (WTTC) has also been set up in Boston, Massachusetts, together with the Massachusetts Clean Energy Center (MassCEC), which can test blades up to 90 m. The WTTC has three test stands for testing.
A full fatigue test usually takes 3-4 months, whereas a static test takes 1-2 weeks. The WTTC has an annual capacity of 6-9 blades a year, whereas the Colorado facility can do 3-4 megawatt (MW)-scale blades a year.
Although testing facilities cannot reveal results of individual tests, they do cooperate on testing methods through proficiency testing needed for accreditation. There does not seem to be much competition between full-scale structural testing centres, as they use fairly similar methods. Manufacturers tend to choose whichever facility is most convenient based on location.
Setting the standard
Narec performs tests in accordance with IEC and ISO standards, as well as customer-specific requirements. The existing 50 m blade test facility is accredited to ISO17025 by the United Kingdom Accreditation Services (UKAS). The new 100 m facility is expected to receive the same accreditation once operational.
NREL is accredited by the American Association of Laboratory Accreditation (A2LA) and tests to international standards from the IEC and also ISO 17025.
Scott Hughes Test Methods Supervisor at NREL's Wind Research Centre, says: “Wind turbine blade testing is governed by the IEC 61400-23 standard. While it will be a relatively new standard it has been a guideline for some time, but recently they have put forth what's called a committee draft for vote (CDV) of a standard for blade testing. We expect the standard to be ratified sometime in April next year.”
There are several certification bodies, and “they all have slightly different requirements for what they think is safe,” Richard Court, Wind Blade Technology Specialist at Narec, says.
“For instance, Germanischer Lloyd's guidance on wind turbines doesn't require a fatigue test on blades – you can do one, but it is not a requirement; whereas DNV and the Danish Wind Turbine Certification Scheme do require a fatigue test. This leads to subtle differences in blades: German blades designed to GL standards tend to be a bit heavier whereas blades that have got a fatigue rating are a bit lighter.”
The fatigue test can give manufacturers the confidence to reduce some material, lowering costs.
Paul Hayden, Chief Technology Officer, at wind turbine blade designer and manufacturer Blade Dynamics, adds that as blades evolve in terms of materials, sizes and the environments they are deployed in, standards and certification bodies will have to adapt.
“It becomes quite hard for one certification body to write one set of rules that covers everything, because there are so many variations,” he says.
Hayden believes certification bodies will need to work even more closely with blade designers.
Will it break?
Whichever the certification body, most blades will undergo a static strength test. This involves putting predefined loads on the blade in several directions, Court explains.
The Massachusetts Clean Energy Center’s (MassCEC) Wind Technology Testing Center (WTTC) offers a full suite of certification tests for turbine blades up to 90 m. WTTC also offers the latest wind turbine blade testing and prototype development methodologies for onshore and offshore blades.
Specifications:
|
“We're checking whether the blades are getting the right strain levels and deflections, and whether they are what the manufacturers expected. Sometimes the test is used to get certification of a blade, and sometimes it's just used by manufacturers to check they are on the right track before making 1000 of them.”
At Narec, the static test is performed using electric winches and cables that are attached to the blade at non-critical points, moving the blade tip through a deflection of 10 m or more. The blade is secured using the same bolts at the root of the blade as would be used when the blade is installed on a wind turbine.
“We usually put around 100 strain gauges on the blade – both on the outer surfaces and maybe a few gauges on interesting internal parts of the blade," Court explains. "We use the standard foil strain gauges and some people are now using fibre optic strain gauges – either bonded onto the surface or occasionally embedded within the blade.”
“We do deflection measurements using either lasers on targets on the blade or spring potentiometer to measure how far the blade has deflected. Sometimes we do that in two directions at the same time, so measuring 'X' and 'Y' – both how far across the blade is going and how much up and down it's going. This is again to check it's performing how you would expect.”
Blade Dynamics' Hayden adds that in static testing, blades undergo loads beyond what you would expect under normal operating conditions.
“For example, if the blade gets hit by a very high gust of wind – the sort you may get once every 50 or 100 years – the blade has to be able to withstand that kind of load. Also, if a rotor is in normal operation and then the emergency stop is activated on the turbine, the rotor comes to a very quick stop – you can imagine it’s a bit like a whiplash type of effect on the blade. You can try to simulate those kinds of loads.”
“Typically with static testing, you simulate the worst load cases and the worst load directions on the blade,” he adds.
The stamina test
After the static strength testing, blades may undergo a fatigue test, which can last 3-4 months.
“The fatigue testing is an approximation of what the turbine blade would receive during its life," Court says. "They push the blades through about one million cycles in the flap and edge directions, trying to simulate what the blades see in real life. It is a very coarse approximation to reality, but it's better than nothing.”
Static testing:
Blade fatigue testing:
|
NREL's Hughes explains further: “The 20 year operational loads you may see on a turbine, depending on the scale of the turbine, are between 0.5 billion and several billion fatigue cycles. What we have to do in the laboratory is take this load spectrum – the in-field load spectrum – and condense and accelerate it to a fatigue load that we can apply in a reasonable amount of time in the laboratory, and that's typically somewhere between one million and five million cycles.”
Fatigue testing moves the blade through quite large deflections – particularly in the flap direction. A 40 m blade may be moved +4 m to -8 m at the tip, putting particular strain at certain points of the blade, putting the blade into motion, making the blades reach their resonance frequency. At Narec, this is done using hydraulic actuators mounted on the blade.
“We put mass on the hydraulic actuators and then move them up and down at the resonance frequency and the blade gradually increases the amplitude it bounces at," Court explains. "As we wind the amplitude of the movement of the actuator up, the blade starts moving more and more. When we reach the target strain level at a particular point – we hold it at that amplitude and the blade gets push along one million cycles.”
The strain on various points is monitored to make sure it is not overloaded.
At NREL, they have developed and help commercialise linear resonant excitation systems.
“In essence, it's a linear oscillating mass that is attached to the blade. The oscillating mass is controlled by a hydraulic actuator,” Hughes explains. “We use a linear hydraulic method which causes a hydraulic cylinder to oscillate the mass in one or more directions, and that's what drives the blade test setup to be excited at the system's natural frequency. By increasing either the mass or the stroke of the actuator, we can increase or decrease the moment that is applied to the blade, tailoring the moment from root to tip.”
The blades are also weighed to check the centre of gravity. The blade's natural frequency is also recorded and checked against the frequencies of operation of the full turbine so you do not get resonance excitation when the blade is in operation. The frequencies are measured using excite-monitors in the blade.
After completed fatigue testing, the blade is usually sent back to recheck static strength in three to four different directions.
Data analysis
Although blades can break during testing, the centres look for more than just a clean break. The blades are analysed to check for cracks, de-laminations and other structural damage.
“The typical measurements that are made in the course of a test are load, deflection and strain," Hughes explains. "Load is measured as you need to validate that the load that you are applying, both statically and fatigue, meets the design objectives. Deflection is measured to ensure that the blade does not lose stiffness during the course of the test. And strain is measured to measure the local properties of the blade and ensure that there's no permanent deformation of the structure occurring during the course of the test.”
There are also other non-destructive evaluation systems.
“These include acoustic emission, which in essence are microphones that are glued to the blade. Acoustic emission systems, in principle, can detect changes or damage occurring to the laminate through cracking or de-lamination of the laminate of a test in progress. Ultrasonic methods are used to check the integrity above the laminate and adhesive joints – between the laminate skin and the shear webs. Infrared inspection can be used passively or actively to inspect the laminate quality and the bond line integrity.”
Not much has been done, however, on x-ray or other high frequency wave-type inspection systems.
An average 45-50 m blade weighs somewhere around 8000-12 000 kg, which means huge amounts and volumes of materials to test, and it becomes impractical, if not impossible, to check every millimetre of a blade. The testing therefore remains at a macro-level unless the blade is damaged.
“Post mortem, in cases where damage has been observed, we will do inspections or actually cut a section of the blade up into smaller components to look and investigate it," Hughes says. "In some cases there are sections of blades that are cut out, sections of laminate that are used for further validation, further testing on a load frame or a subcomponent rig.”
“Otherwise, when you try to look at more of the structure, you use techniques such as full field strain where you apply patterns to the blade and use image correlation to look at a surface or systems such as acoustic emission to detect damage. There is quite a considerable amount of visual inspections that go on during the test and between in load cases and before, after and during fatigue testing. You also use visual observation to locate damage sites. However, fundamentally, it is a challenge to be able to quantify the full volume of a wind turbine blade.”
Once the data is collected, however, there is no official statistical background to lean upon when analysing results. Typically, only one – maybe two – samples of a given blade design is tested due to economic and time considerations.
Blade confidential
Furthermore, each blade manufacturer does things slightly differently and the testing facilities are usually put under strict confidentiality agreements.
Narec's Court explains: “It is hard won information because the manufacturer has probably tried two-three things in the past that haven't worked particularly well, and you often only find that out by making the thing and then testing it."
Blade Dynamics' most recent blade is the D49 hybrid made of epoxy, glass fibre and carbon fibre, with the company's Bladeskyn surface coating. The 48.63 m long blade weighs 5900 kg and has been designed to work especially well with AMSC's Windtec 2 MW wind turbine. The turbine blade manufacturer currently has a blade undergoing testing at the WWTC in Boston, MA, USA. The company has its blades tested to GL certification standards at the moment, but does not exclude the possibility of using different testing bodies in the future depending on their expertise on testing for different environments and applications. |
“If you look at the various manufacturers' blades, they are different inside in terms of the solution they've come up with. Some people have i-beams, some have box beams, two-spars, some have integral things, some have things they bond on later, and that's all their clever secret bit. We know that, because we see inside the blade.”
But the inside knowledge is not to be shared due to intellectual property and commercial concerns.
“We're doing some work with European funded programmes and the work from that is publicly available, but it tends to be very generic," Court says. "Because it always comes down to the detail of a particular design, that particular design is not really applicable to anybody else's. That information is the intellectual property, and paid for expense, of that company. They are not going to share that willingly.”
This means there could be times when a testing facility will look at a blade and wonder about design choices, but as Court says: “you do the test and then that information gets found out by that particular customer. And blades do sometimes behave unexpectedly – we do have things happening where we say: 'we didn't expect that' – nor does the customer.”
Materials and environments
Although full-scale blade testing methods do not vary much depending on materials and whether the blade will be used on- or offshore, Narec's Court says the difference between onshore and offshore blades is not that great at the full-scale level, but adds that “the interesting thing is done in the manufacture of the blade and the manufacturers' choice and cleverness in use of materials and design of structures – and combining that with airfoils.”
Nevertheless, the choice of materials has some impact. Carbon, for example, is lighter and stiffer. “That makes it easier to handle in our lab,” Court says.
As blades get bigger, the more common glass fibre blades are causing some issues for testing facilities.
“We find that as the blades get longer, we've had to build a new facility, because we cannot test them in our old facility – it is not wide enough. We've had a direct impact on the manufacturers not using carbon.”
Court believes the price of carbon means many manufacturers still use glass fibre even as blades get larger, “but they are a bit more bendy and we've had to invest money in a facility that can cope with that.”
NREL's Hughes adds: “The inverse slope parameters, which is a principle metric to quantify fatigue life, vary between materials. Fibreglass composites may have inverse load parameters of 8-12, the carbon will have an inverse load parameter of 14. ... So the acceleration of the loads for fatigue testing is a little different between fibreglass and carbon.”
The load parameters are usually determined by the manufacturer at coupon level testing, and this data is then used in the fatigue testing to set up the test load.
There are no specific standards for testing offshore blades, but NREL is looking into it as the US prepares for its planned offshore wind developments.
“This is something we're getting our feet wet on – to use the pun,” Hughes says. “Some of the work that is going on now is trying to address this for a comprehensive test programme.”
He adds: “Currently, the full-scale testing that we do is the same between onshore and offshore, but that may change in the future.”
Wind turbine blades also undergo lightning protection tests, but this is mainly done in electrical continuity testing. The main difference here is that glass fibre is a relative insulator, whereas carbon is a conductor, which requires slightly different systems and testing.
Manufacturing 'secrets'
Whereas the test centres focus on full blade testing, coupon testing is done by blade companies in both their own and external laboratories. Extensive coupon testing is vital as the full blade test should, ideally, only act as verification.
“As they say in athletics, the race is won or lost before the start gun goes off,” says Theo Botha, Sales Director at Blade Dynamics.
At the coupon level, “you know that the amount of effort you put in is really going to pay dividend when you scale that up to a full blade,” Hayden says.
He explains: “You test to characterise the [tensile, compression, laminate sheer, etc] data so you get some very accurate data to design with. The coupon level testing helps designers choose materials and design structures depending on what effects they look for in a blade – be it extra strength, flexibility, weight or sheer size. For example, for materials where high compression strength is needed, you need to hold the fibres in column, so therefore you need to make sure the fibres are straight – you need to make sure the matrix is as void-free as possible and that you get very few point defects.”
Materials undergo different tests depending on what the blade will consist of, and where it will be deployed.
“Your wind turbine could be designed for cold climate conditions, so there are some specific examples where you would want to do certain tests to check for example phase changes of the resin at the lower end. It could also be at the hot end – you would want to check that the material isn't going to go soft when it gets very hot."
“Then for offshore environments, you've got continuous exposure to salt water. Sometimes that can affect the properties – it can affect how the density of the laminate evolves over time.”
For offshore turbines, the blade material would be subjected to accelerated salt environment testing and surfaces undergo ultraviolet (UV) testing, Hayden explains.
One of the challenges is to make sure the coupon level sample is representative of what is actually going into the blade.
“Typically with key structural materials like unidirectional carbon fibre material, the coupons are usually results on the low side because you get edge effects and damage on the test surfaces of the coupons – that's normal. But if you do a statistical analysis on it, you can normally show that what you get in the bulk structure of the blade itself would be better.”
“Coupon testing is really the area that a lot of blade manufacturers are now really focusing on, because the better you’re testing, the better your design numbers, which allows you then to use more effectively material within the blade.”
It is also important that the testing allows for the fact that you may get one-off manufacturing defects in the manufacturing process.
“You can easily get yourself in trouble thinking you've got great properties, particularly with carbon, but you only need one point defect to appear in your manufacturing process and that could bring the tip of your blade off pretty quickly,” Hayden says.
It is also very important that the coupon testing is done to as high a degree of accuracy as possible. Hayden says there can be quite large differences between facilities: “If you, for example, have got a mechanical testing standard for tensile testing of a unidirectional composite laminate, you can make the exact same samples and send them to three different test labs and they would all come back with different data. So choosing where you do your testing makes a big, big difference.”
Blade Dynamics has found it gets the best external coupon testing results using facilities in Japan because they take great care with the samples and tests.
“It's a big issue trying to find consistent testing across the board and the current standards in place don't really do that. This encourages people to do a lot more in-house testing, because at least they get some kind of control over the consistency themselves,” Hayden says.
The missing link
There are centres for full blade testing and laboratories for coupon testing, but what about the intermediate step?
“Usually, during the design process, there's quite a lot of testing that goes on at a coupon level, quantifying materials – resins and fabric at a small level," NREL's Hughes says. "But we step from coupon level testing to full-scale level testing with no real intermediate testing as is done in aerospace, automotive or infrastructure fields. That is one aspect that probably could be improved upon as the introduction of intermediate scale testing where you are able to test smaller, but representative articles to gain more confidence in the lifetime durability of a blade.”
Hayden at Blade Dynamics agrees: “One of the short-comings of testing is that it is easy to make nice little coupons or small samples that you can test in a laboratory. There's a big difference between that and the type of variation you get when you manufacture something bigger, like a wind turbine blade.”
He adds: “With a full blade test – at that time you're hopefully not testing to learn something, you're just trying to validate that what you designed does what you want it to do, because it's quite an expensive test and quite a big undertaking for a company to do a significant amount of large structural testing."
“If you are able to test something in the middle, sub-components for example, you can get a lot more quality assurance because you're generating more data on a more representative scale. This is something we've been working quite hard on – to do better testing and more of it in a more cost effective way so that we can give customers more assurance on the quality of the blade."
Another way to validate design and materials is to develop and test smaller scale blades that can be representative of the larger ones, Hughes says.
NREL's Scott Hughes explains the most common laboratory test inspection methods. Ultrasonic methods are widely used on composites including applications such as pipelines. Typically, pulse echo ultrasonic methods are used for wind turbine blades due to a lack of access inside the blade towards the tip. In the root area, pitch-catch ultrasonic methods can be used as access to the inside of the blade in the root area. Systems can be calibrated for depth so you can look into a laminate to see if there are voids or de-laminations. However, one runs into problems at the root of the blade due to the thickness of the material – you cannot peer through the composite. The acoustic emission system consists of high-frequency microphones that are glued to the blade. This is an active method for damage detection. If you have a damage occurring in the blade such as a propagation of de-lamination or a bond line cracking between web and skin, or at the leading edge or trailing end of the blade, the microphones pick up the acoustic energy that is emitted by the damage. By arranging the acoustic emission sensors on the blade, you can use triangulation to detect where the damage is occurring. The calibration is calculated depending on the speed of wave propagation. Composite materials have different material velocities, so the speed by which the waves travel along the blade or in the cord-wise direction of the blade varies – also depending on the materials. The materials and fibre directions also vary depending on the location within the blade, and so the speed of the wave propagation varies considerably over the volume of the blade. A basic system can be purchased for around US$15 000 and the more sensors, the higher the cost, but the acoustic emission method is nevertheless regarded as fairly inexpensive compared to many other methods. Infrared or near-infrared is used to sense changes in temperature. It can be used for initial inspections of the blade by applying heat or cold to the cavity of the blade and then imaging the blade from the outside. What you're looking for are areas where you have a temperature radiant – so if the bond line is not complete, or there are voids, this will be indicated on the outside surface as heat (or cold). It can also be used actively during fatigue testing as areas experiencing damage are going to be hotter than surrounding areas. When fabric rubs or an adhesive is broken, thermal energy is released, which can show up on an infrared camera. It is most useful if the damage is near either the inside or the outside skin, as glass fibre is very insulating, temperatures have often evened out by the time the thermal energy from damage gets to the surface. |
“We do quite a lot of R&D work on 9 m blades. The large 45 m blades might cost somewhere between US$100 000 and US$200 000 – the metric for making a blade is around US$5-6/lb, so for testing programmes where we're trying to look at effects of only testing one or two blades instead of doing a statistically based test. Also if we're testing out a novel design feature like bend-twist coupling where we're introducing a passive load intimation to the blade by angling fibres or adding sweep to a blade."
“Starting around 9 m scale you can build a representative structure, test on it and then do scaling studies and concert with that for larger structures. So we have a $5000-10 000 test article instead of a $100 000, or likely more, test article.”
Bigger and bigger
“As blades get bigger, it takes a lot more time to test them, and can be a lot more costly to the manufacturers,” Paul Parry, Commercial Manager at Narec says.
Court adds that it also gets more difficult to test and analyse larger blades. Bigger blades also take up a lot more space.
Parry says: “The longer the blades, the longer the deflection – because a lot of these blades are quite flexible.”
This is the reason Narec is investing in its new 100 m blade facility. Other than size, however, the facility will not be very different from the current blade test stand.
“The new facility is basically a scale-up of what currently exists.”
The blades to fill these larger facilities are already being made. 60 m blades are in production and 70 m blades are in preliminary production for the new 6-7 MW wind turbines. Court says there are even programmes around asking people to design 90 m blades. He does not believe blades exceeding the 100 m capacity of the new facility are likely to come in the near future. Either way, he thinks the testing of such large blades would be too costly as the tips could move 25 m or so before failure, which would require huge testing facilities.
The increasing sizes of wind turbine blades are also posing some problems in terms of inspection and analysis. Court explains: “The inspection of such large structures is a massive problem, no one really knows how to do it economically and affordably, so that's why the inspection we do is mainly visual. We hope to see the damage if it occurs, but if anyone comes up with any techniques ... rapid, cheap, large-area inspection would be a major breakthrough. But if you look at all the available technologies, I'd be intrigued to see what that could be.” ♦
This article was published in the January/February 2012 issue of Reinforced Plastics magazine. To apply to receive your free copy of each issue of Reinforced Plastics please complete the subscription form.