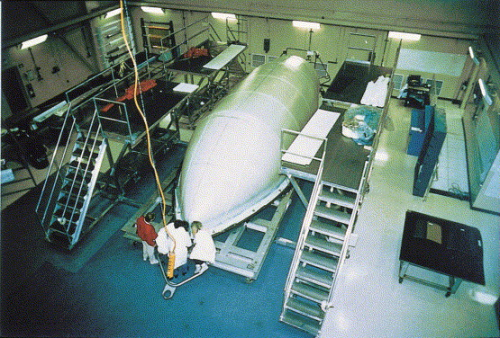
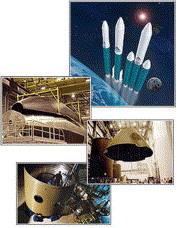
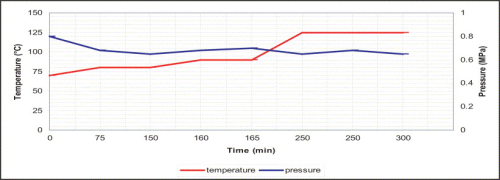
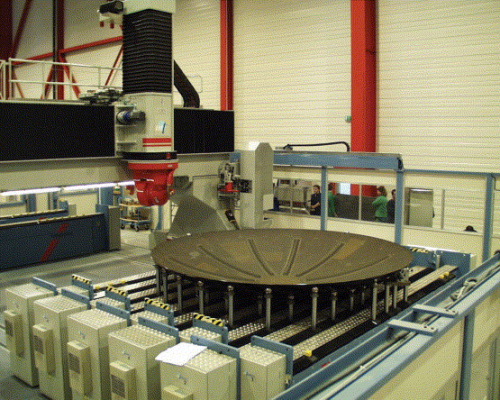
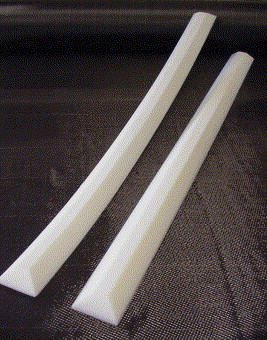
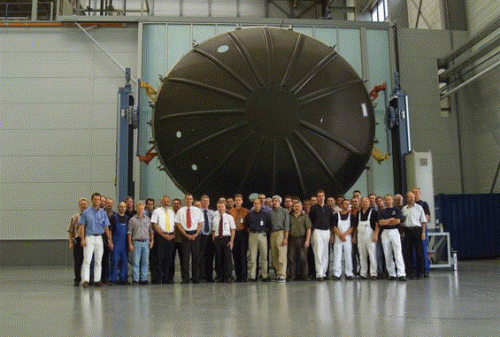
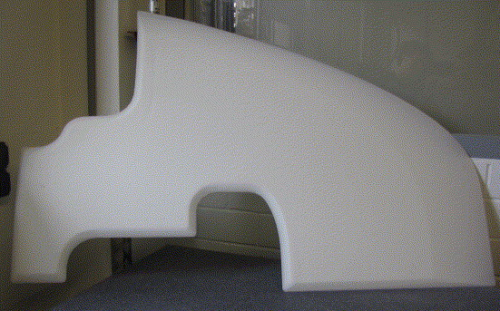
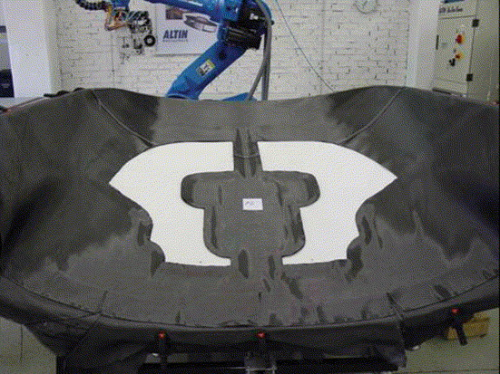
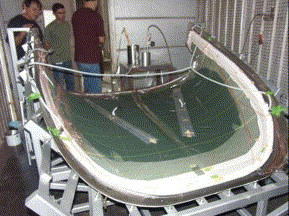
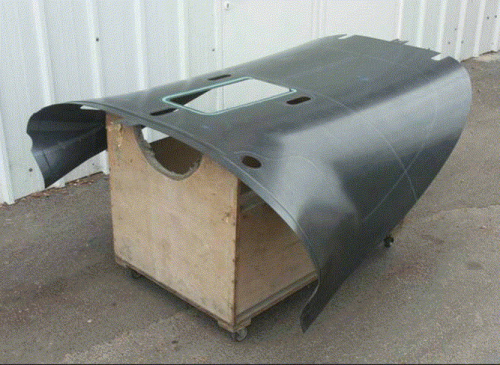
The history of aerospace applications using polymethacrylimide (PMI) foam started in 1971, when the material was successfully introduced as structural sandwich core for helicopter fuselage panels. Today PMI foams are listed in more than 170 aerospace specifications worldwide.
Sandwich technology contributes significantly to solving weight problems in many fields of application. If we focus on the manufacture of aircraft components, mainly Nomex® and aluminium honeycombs are used as core materials. They offer excellent strength-to-weight and modulus-to-weight ratios. On the other hand, the non-isotropic and open-cell character of honeycombs causes some problems during core shaping and curing. Honeycombs do not withstand lateral forces/pressures and tend to collapse during core shaping and sandwich cure. They do not fully support prepreg layers during co-curing operations, which results in surface imperfections and zones of poor laminate consolidation and fibre disorientations. Another major problem is extensive moisture absorption and corrosion, which limits the performance of the component and causes increased expenditure for repair and higher service life costs. For these reasons, suitable rigid foam cores based on PMI have been utilised in manufacturing sandwich components for aerospace components for quite some time now. This includes secondary structures as well as primary structures for state-of-the-art aircraft.
Delta 4 satellite launch vehicles
The idea of using PMI foam in the design of the Delta 4 sandwich components leads back to the design of the composite payload fairing for the Delta 2. The goal for the new development was cost reduction in the manufacturing process as well as in the assembly of the fairing. PMI foam was used as a bi-functional core serving as a mandrel and as a structural member of the sandwich contributing to buckling resistance. The decision was prompted by the fact that PMI foam can easily be thermoformed to even very complex geometries such as the nose section of the fairing. This makes it possible to implement a simple male tool plus a cost-saving lay-up and cure procedure. Prepreg for the inner skin of the component is laid up first, followed by the precisely thermoformed patterns of PMI foam, forming the mandrel for the lay-up of the outer prepreg skins. The entire lay-up is vacuum-bagged and co-cured at 175°C in one shot (Figure 1).
The excellent resistance to compression creep of the PMI foam, plus its ability to fully support the prepreg during the cure results in a high dimensional stability plus a perfect consolidation of the prepreg. Compared to an aluminium honeycomb-cored design the surface does not show any dimpling effects and no local zones of lesser compaction of the prepreg or micro fibre disorientation. Using the PMI foam core gives much better consolidated skins and the same level of buckling resistance can be achieved with a smaller numbers of prepreg layers. This means additional cost savings.
As a result of the excellent experience gained from the Delta 2 fairing re-design PMI foam-cored sandwich has designed into a number of additional structures for the Delta 4, Delta 4 Medium and Delta 4 Heavy models. PMI-cored sandwich accounts for 40% of the structural parts of Delta 4 Heavy (Figure 2).
Japanese H2A satellite launcher
The National Space Development Agency of Japan (NASDA) approved a PMI-cored sandwich design for the interstage of the Japanese H2A rocket. The large structure is manufactured by MHI, Nagoya, using the cost-effective co-curing technology.
Similar to the manufacturing of the Delta 4 cylindrical sandwich structures, a large aluminium barrel mould is used. The inner layers of carbon/epoxy prepreg are placed on the mould first, followed by a layer of thermoformed and net-shaped and butt-joined PMI foam segments. As a last step the outer layers of prepreg are placed on the PMI foam core, acting as a mandrel and as a structural member of the sandwich design. The completed lay-up is vacuum-bagged and co-cured in the autoclave.
Rotor craft
In 1971 ROHACELL® PMI foam received the first aircraft specifications for the Eurocopter Ecureil EC120 helicopter. The continuing success story of the use of high performance PMI foams in numerous helicopter models started with applications in the fuselage and in the main rotor blades of this helicopter platform, which is still being built in significant quantities.
Today, the manufacturing of high performance advanced helicopter rotor blades using Rohacell structural sandwich cores is state-of-the-art technology. The initial change from old metal blade design to composite blades was prompted by the wish to solve corrosion problems and the demand for increased performance and service life of the blades. A manufacturing method using PMI foam cores as an ‘active’ mandrel and structural part of the component was developed in close cooperation with a major European helicopter manufacturer and the Rohacell engineering team. The technology is well known as the in-mould pressure process. During the closed mould curing process the thermoelastic behaviour of the foam core provides internal pressure, perfectly consolidating even massive prepreg layers against the mould surface.
Owing to the excellent creep compress-ion resistance, such foam cores can provide a very high and constant level of pressure. In mould pressures of up to 7 bars can be reached and maintained during a 2-hour cure cycle (Figure 3). The desired peak pressure can be adjusted to precisely meet the gel point of the epoxy prepreg. The high level of mechanical properties of the foam improves buckling resistance of the mostly very thin U-spars used in the blade design. Due to its outstanding fatigue behaviour, which is better than any other rigid foam, PMI foam withstands the high dynamic loads to which the rotor blades are exposed during their service life.
By using the Rohacell foam-cored design, the service life of rotor blades was increased from approximately 400-500 flight hours for the initial metal blade, to more than 10 000 flight hours today. A detailed study carried out by Bell concluded that the manufacturing costs for a PMI foam-cored tail rotor blade are only about 20% of the costs for a honeycomb-cored version. The reason for this is that the foam core makes it possible to introduce a single curing process, whereas the honeycomb-cored version requires several curing and bonding operations. In addition, machining of the foam core is much easier and up to 10 times higher feed rates are possible.
PMI foam cores have been successfully introduced in many major blade programmes. The main blade of Westland's EH101 helicopter is probably the most impressive example. The length of the blade is approximately 8.5 m.
Eurocopter makes intensive use of PMI foam cores for their range of helicopters. Today approximately 1800 individual parts of the BK117, EC 120, EC 135, Tiger and NH90 use Rohacell foam cores. A number of Asian-built helicopters, such as the Indian Advanced Light Helicopter (ALH), and the Japanese MH 2000 built by MHI and the OX 1 built by KHI, have also adopted the PMI foam-cored rotor blade design.
Civil aircraft: stringer stiffened applications
As a result of a successful cooperation with Airbus Deutschland GmbH, Werk Stade a new composite concept for the pressure bulkhead of the Airbus A340 (Figure 4) and A380 fuselage was realised. The carbon/epoxy shell structure is stiffened by A-stringers filled with a core of Rohacell 71 WF-HT. More than 1200 thermoformed and ready-to-use stringer profiles for the A340 have already been delivered to the Airbus Stade plant. Nine stringer profiles are used per bulkhead. The complex shapes are manufactured and delivered ready-to-use including comprehensive quality assurance (QA) documentation and incorporated test coupons (Figure 5).
Based on the good experiences gained from the A340 project, the new design of the bulkhead using Rohacell-filled stringers was adopted for the A380 super widebody (Figure 6). The dimensions of the stringers are even bigger and, due to a twist, the shape is more complex. The 2.5 m long stringers are also precisely machined and thermoformed to conform to the contour of the bulkhead. 15 of the foam core profiles are used for one of the impressive bulkheads measuring approximately 6 m in height and 5 m in width. In the Airbus application the foam is only used as a mandrel, however strength and stability of a carbon/epoxy shell structures can be significantly improved by using A-stringers stabilised by a structural PMI core. The foam-stabilised stringer outperforms the hollow stiffener by far, because the failure caused by stability is almost moved to the yield strength of the structure. This makes it possible to design the stiffener profile with minimised wall thickness, resulting in a significant weight reduction. The achievable level of optimisation is dependent upon the actual geometry of the stiffener profile and can reach 40% improvement regarding the failure load. The level of stability can even be improved by up to 100%.
Civil aircraft: structural parts
The horizontal stabiliser of the Embraer 145 aircraft is realised by the cost-effective co-curing process using a high performance PMI foam core. The part is cured at 180°C and 0.35 MPa. No other foam core with a density of only 70 kg/m3 would withstand these cure parameters. The foam acts as a mandrel and it is a structural member of the sandwich design.
The new design of the landing gear doors of the Dassault Falcon 7X is realised by resin transfer moulding (RTM) using RTM6 resin. Due to the injection and post-cure parameters the choice of structural sandwich foam core is the XT (eXtended Temperature) version of PMI. This particular core material can take curing temperatures of up to 190°C and pressures of up to 0.7 MPa during processing. Post-cure temperatures of up to 225°C can be applied.
The recently rolled out Gulfstream G150 is fitted with a new generation belly fairing realised by means of vacuum assisted resin infusion (VARI). A pre-net-shaped core of PMI foam is precisely thermoformed to the required contour (Figure 7). In the next step dry reinforcing material is stitched to the core making a ready-to-use assembly which is easy to handle (Figure 8). The assembly is put on a female tool and is vacuum bagged. Resin is infused by means of vacuum suction and the part is post-cured after the injection process has been finished. The rigid foam core acts as a mandrel, carrying the dry reinforcement and is also a structural member of the sandwich design. The parts produced by the low-cost VARI process have excellent surface finish and the skins have a very high fibre volume fraction (Figure 9 and Figure 10).
Benefits
In conclusion it can be stated that high-performance PMI foams meet the demands for autoclave and any kind of resin infusion processes including high performance RTM particularly well.
The excellent creep compression resistance and outstanding temperature resistance of PMI foams make it possible to use them for all common cure cycles. Post-curing temperatures up to 225°C are allowed.
Although honeycombs offer a superior strength-to-weight ratio, PMI rigid foam cores are a good alternative if cost-effective co-curing is performed and some additional weight can be tolerated. If a particular honeycomb-cored component requires the use of certain amounts of epoxy potting, the honeycomb sandwich version can even be heavier than the foam-cored version. Beside the obvious cost savings in core shaping and processing, it has already been proven that PMI-cored panels lead to reduced life cycle cost. The reason for this is the significantly lower moisture uptake of PMI-cored sandwich panels compared to the honeycomb cored design. Depending on the actual application and the design, significant improvements in impact resistance and compression after impact (CAI)-behaviour can also be realised.
This paper was presented at the RP Asia 2005 conference held in Bangkok, Thailand, on 25-26 August 2005.