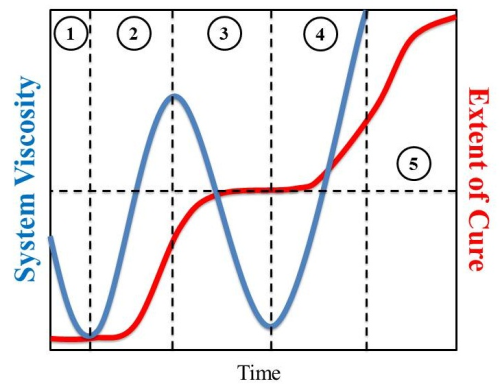
See Part 1 of Lightweighting matters in energy storage
Achieving the right toughness with the right material
A popular choice for wound pressure vessel composite applications is amine or anhydride cured epoxy systems. Epoxy resins are popular because they exhibit high chemical and corrosion resistance, good mechanical properties, good thermal properties, low shrinkage during curing and can be processed under a wide range of conditions. These formulated systems can be modified with a number of additives and fillers to increase toughness. Epoxy cures into a tightly cross-linked structure that provides excellent thermal and chemical resistance. A drawback of this high degree of crosslinking is the reduction of ductility. To counteract the brittle nature of the cured epoxy matrix, toughening techniques have been the subject of wide study [2]. One area of interest is to improve fracture toughness of the resin system through the associated chemistry. An epoxy resin’s resistance to fracture is typically characterised by its K1c fracture toughness [3]. This is the measure of a material, containing a crack, to resist fracture or crack propagation and is one of the most important properties when designing composite structures. The first of two techniques to toughen epoxy resins is through chemical modification. This is done through modification of the cured epoxy matrix to give more flexibility by lowering the crosslink density. Reductions to the crosslink density can be achieved with several techniques, two of which are increasing the molecular weight of the epoxy monomers and/or decreasing the functionality of the curing agent. Another approach for toughening cured epoxy matrices is through the use of some sort of additive. Some efforts at toughening have used carboxyl-terminated and amine terminated copolymer of butadiene and acrylonitrile (CTBN and ATBN), poly(methyl methacrylate) (PMMA) and siloxane rubbers, core-shell rubber, thermoplastics and rigid inorganic fillers such as glass beads and alumina. A second approach for toughening has been through the use of block co-polymer technology [4]. The phase separation of the block co-polymer from the epoxy resin within the resin portion of the composite structure allows for increased deformation before failure through a crack bridging mechanism. Recently, The Dow Chemical Company has introduced VORAFORCE™ formulated systems for composite applications. These systems have been observed to double fracture toughness with minimal change in both processing and mechanical properties. Nanoparticles have recently seen introduction in epoxy resin formulation for increased mechanical properties but have been limited in their uses for filament winding thus far [5]. Fibre manufacturers have also worked to improve fibre grades. For instance, glass fibre manufacturers have designed glass fibre grades which can try to compete with carbon fibre in pressure vessel applications where historically only carbon fibre would be used. These attempts at modifying the glass fibres have been delivering good results and enabling certification approvals, like the EN110 certification for composite tanks for use in vehicles. The use of this high-performance glass fibre in a type IV cylinder gives an intermediate light-weighting effect versus a type IV carbon fibre composite CNG cylinder but with a significant material price advantage over carbon fibre.
Speeding up the production cycle
An equally important area of interest in the manufacture of composite pressure vessels is to decrease production or cycle time. One such method for doing this is by changing the chemistry to incorporate different types of catalysts which can speed cure time through various mechanisms. Previous work has used ultraviolet and thermally catalysed chemistry to speed reaction time but these have seen limited application because of the specific processing requirement inherent to each system. In the case of ultraviolet (UV) curing, the composite system must be thin enough that the UV light can penetrate the entire thickness of the composite to cure the resin fully. When using thermally catalysed systems, care must be taken to control the peak exotherm temperature of the composite so as not to damage the liner. Another recent development to aid in speeding up the process of composite pressure vessel filament winding is ‘towpreg’ which uses a two-stage curing and viscosity profile shown in Figure 4. With this technology the fibres are impregnated with the formulated system at some time before (weeks or even months) the winding operation, partially cured and stored for future use (‘1’, ‘2’ stages in Figure 4). This partially cured glass or carbon fibre composite is then unwound from storage and wound onto the part geometry while being heated. This reduces the viscosity of the towpreg enough for the different fibre/resin tows to adhere to one another (‘3’ stage in Figure 4). Dropping the viscosity by applying heat initiates the latent catalyst driving the viscosity up and the system to full cure (‘4’ and ‘5’ stages in Figure 4). It is easy to see that this two stage process can ease processing through both speed and needed equipment.
Lightweight composites to contribute to sustainable future
With the current interest in exploiting natural gas resources and environmental and regulatory pressure to reduce CO2 emissions of vehicles that requires significant lightweighting steps, global forecasts for CNG (vehicles and associated supporting infrastructure) and also LPG (in particular for the civil use) are very optimistic [6]. To enable large scale acceptance of composite pressure vessels in the market, it will be important to address the industry end-users and their remaining potential concerns, like health in use regulations and end of life assessment and repair. In parallel, it is also important to further influence and improve the regulatory picture, to increase harmonisation and to optimise testing protocols. In particular, within the transportation market current standards still vary widely between geographies and regions in terms of what types of vessels and configurations of vessels are allowed. This makes it complex for the manufacturer and end-users to adopt new and advanced composite cylinder-based solutions. Other storage applications outside LPG and CNG storage are also attracting interest from composite pressure vessel manufacturers. One such technology is the use hydrogen fuel cells and on board storage of the gas. Current storage vessel technology and the production of those vessels is far from ready to satisfy the needs currently targeted by the automotive industry for hydrogen use in motor vehicles. The interest was confirmed more recently from the UK government, which announced the ‘HyFive’ project, where ‘leading motor manufacturers, hydrogen fuel suppliers, Mayor of London Office and energy consultancies from around the globe have agreed to deploy a total of 110 hydrogen fuel cell vehicles at several European locations’ [7]. Another application of composite pressure vessels are in the storage of compressed air. Recently, Lightsail Energy has been using compressed air as a means of storing energy for later use [8]. Finally, the use of conformable composite pressure vessels could play an increased role moving forward in applications where cylindrical geometries are unlikely or not possible. If current trends continue, it will be imperative to maintain a customer-focused mindset to drive market enabled technologies that bring value to customers in the composite market. Moving forward, trends have emerged for composite pressure vessels end-use that provide insight to customers’ immediate needs such as toughening for more diverse application and speed of processing due to market demand. It is essential moving forward that current customer demands are met with innovative solutions while unmet needs are identified, prioritised and solutions are driven to the market quickly.
References
2. McGarry, F. J. In Polymer Toughening, Arends, C. B., ed., Marcel Dekker, Inc.: New York,1996, pp 175-188. 3. ASTM Standard D3039, 2008, “Standard Test Method for ,” ASTM International, West Conshohocken, PA, 2008. 4. Jacob, G.C. et al. “Fatigue Improvements in Toughened Epoxy Systems.” ANTEC Conference and Proceedings (2011). 5. Abrams, Michael “Nanos in the Pressure Vessel.” ASME Online. August 2012. 6. “Growth Opportunities in Global Composites Industry 2013-2018.” Lucintel Report, March 2013. 7. “£2.8m contract to supply three refuelling stations in London.” ITM Power: April 3rd, 2014 (Accessed: April 26th, 2014). 8. Spendlove, T. “Compressed Air Energy Storage - A Moonshot Project.”
See Part 1 of Lightweighting matters in energy storage
This article was published in the July/August 2014 issue of Reinforced Plastics magazine.
The digital edition of Reinforced Plastics is distributed free of charge to readers who meet our qualifying criteria. You can apply to receive your free copy by completing this short registration form.