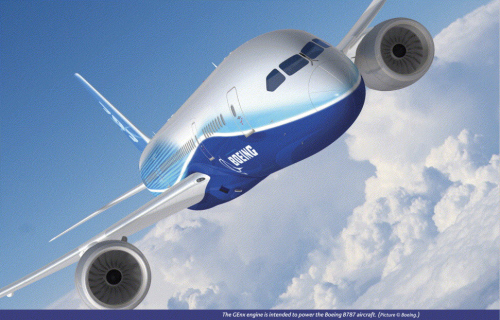
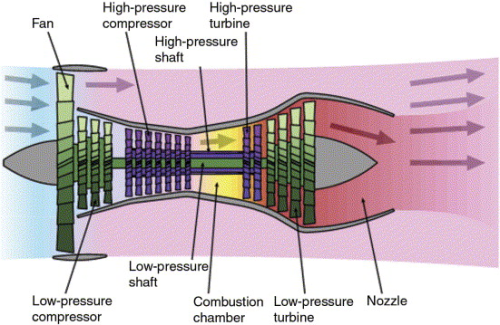
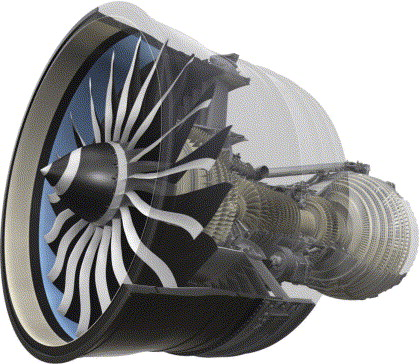
On 19 March 2006, in Peebles, Ohio, a new jet engine ran for the first time. Superficially, it looked and sounded like many other jet engines for passenger planes but, in fact, the GEnx (General Electric next generation) heralds a radical advance in aero engine technology. This is the only jet engine being developed with both fan case and fan blades made of composite materials, a breakthrough that provides significant weight reduction, reduced fuel consumption and enhanced engine durability.
These gains are fully in keeping with the platform the GEnx is intended to power, the Boeing B787 airliner, which is now in production development. With luck and care, this aircraft will show that reinforced plastics are suitable not only for the most critical primary airframe structures of commercial airliners – most of the 787's airframe, including the fuselage, being made from composite materials – but also for the rotating innards of their engines.
Engines and composites are no strangers to each other, though these versatile materials have so far been best known for their weight-saving contributions to nacelles, thrust reversers, ducts, guide vanes and other items outside the engine proper. But designers have for some time wanted to extend the benefits to critical components inside the engine. Polymer composites are temperature limited and therefore unsuitable for use in engine hot sections, but this is not the case for the cold sections. The item designers consider could most benefit is the large fan found at the front of turbofan engines that power the airliners of today.
Blades
Blades on fans in current-generation turbofan engines can experience centrifugal loadings of around 100 tons – equivalent to the weight of a diesel locomotive hanging on each blade. The GEnx fan will be large, with a diameter of 111 inches, so that a high by-pass ratio, 9.5:1, can be achieved, enhancing engine efficiency and reducing fuel burn. It will revolve at some 2500 rpm in the cruise. Centrifugal loading increases with radius, with rotational speed and with blade mass. Radius and speed are determined by airflow considerations, but anything the designers can do to reduce blade mass will reduce loadings – hence the switch in certain GE engines from conventional hollow titanium blades to composite. This provides a 10-15% weight reduction, according to Melvyn Heard, marketing manager for the GEnx programme.
Lighter blades permit weight savings elsewhere in the engine. Reduced internal stresses within the fan assembly mean that it can be less highly engineered for strength and therefore lighter overall. More significant, however, is the issue of containment – the need to contain within the engine space any blade that detaches, flies off and might otherwise penetrate parts of the aircraft including fuel tanks or the passenger cabin. Blade separations are rare, but can be precipitated by an internal defect or ingestion into the engine of foreign objects such as birds, or ice cubes in a hail storm. Containment is the role of the fan containment case, a surrounding sleeve designed to arrest the progress of high-speed debris. Traditionally, metal fan blades have required predominantly metal containment sleeves, but lighter composite blades have less energy and can be stopped by all-composite cases that are substantially less heavy than metal.
This, therefore, is a happy instance in which one composite application, if achievable, can lead directly to another, the two being complementary. GE believes that it has achieved this double composite gain, its reduced-mass blades and case together saving some 350 lb in weight, or 700 lb in a twin-engined aircraft. Actually, the saving is even greater than this since lighter engine parts mean that designers can avoid weight in other parts of the installation.
Blades represent, however, a tough material challenge. They must, as already mentioned, resist high centrifugal loads, without distorting or ‘creeping’ over time. Creep cannot be tolerated because the clearance between blade tips and the duct inside which they turn have to be minimal for reasons of operating efficiency, and any outward migration of the tip would bring it into scraping contact. Blades also face rain, hail, ice, repeated thermal cycling across a high temperature range, icing, foreign object ingestion and attack by airborne chemicals. One of the tests aero engines are subjected to during certification trials is the ‘chicken gun test,’ when bird carcasses of various weights between 2.5 lb and 8 lb are fired into operating engines. It is the fan that first has to resist the consequential effects.
First
The quest for composite blades in fans and compressors is several decades old. The first aero engine to have composite compressor blades was the Rolls-Royce RB108, designed in the early 1950s. These blades were of GRP. Much later, in the early 1970s, Rolls-Royce used composites in a turbofan engine. Fan blades for the RB211-22B were of ‘Hyfil’, a carbon fibre reinforced epoxy. The blades were of a new wide-chord design pioneered by the company to reduce the number of blades necessary, and hence cut noise.
Unfortunately, Rolls-Royce had problems with the RB211, a number of these being associated with the composite blades, which lacked, in particular, adequate bird strike resistance and manufacturing repeatability. Engineers had perhaps placed too much confidence in the use of a material which, at that time, was unproven for major rotating parts and, while they had a sound understanding of the material's properties at laminate level, this did not extend into the highly dynamic environment of a fast-rotating component. The problem could not be solved in the time available and Rolls-Royce had to switch back to titanium blades. The RB211's troubles contributed to the 1973 bankruptcy of the company.
Nor did General Electric avoid trouble with its own first composite fan blade venture when, in 1971, it produced carbonfibre blades for TF39 engines powering the US Air Force's C5 Galaxy heavy lifter. Not until the mid 1980s did the US company reckon to have fully acceptable blades, and these were produced for the GE36 unducted fan project, though this died for lack of airline interest.
By the mid ‘90s however, the company was ready to launch a composite bladed fan on the GE90-76B engine selected for the Boeing B777 widebody twinjet. By then it was using three-dimensional (3D) aerodynamic modelling to evaluate designs without having to demonstrate them in full-scale testing at every design iteration. These methods were used in developing blades of strongly swept wide-chord form that were highly efficient, enabling far fewer blades to be used than hitherto. The GE90 fan has 22 blades compared with 36 on a typical current-generation engine, GE's CF6 model. Composites were a key enabler for these advanced new blades.
The GE90 became the only commercial turbofan using a primarily composite fan blade, and success in operation earned the blades a place in subsequent marks of the engine too. Years of effort and investment aimed at bringing composites into engine interiors were beginning to pay off.
A modern jet airliner engine has at its front end a large fast-rotating fan, the outer part of which is effectively a ducted propeller while the inner part is a low-pressure compressor, compressing air that will enter the combustor at the engine's core. Much of the air entering the engine's air intake will pass through the outer part of the fan, by-passing the engine core altogether. This air will emerge at the engine nozzle, co-axially with the high-velocity jet emerging from the engine core. The overall effect is to develop the required total thrust at modest velocity over a large cross-section area, rather than from a highly concentrated high-velocity jet alone. This improves fuel efficiency at conventional aircraft cruise speeds, and greatly reduces noise. Commercial turbofan evolution has been marked by ever greater volumes of air by-passing the core – giving higher by-pass ratios and greater operating efficiency. A contemporary turbofan powered aircraft can therefore be said to be as much propeller (fan) driven as jet propelled. The fan/compressor/propeller is driven by energy extracted by a turbine downstream of the combustor in the engine core (see diagram). Weight reduction is always a strong driver in aeronautics and a set of fan blades that is lighter than any that existed hitherto will automatically help in producing lighter engines that reduce fuel burn while increasing payload and range. However, the dynamics of a fan multiply the weight-saving significance several times over. |
GE90 blades are made using preforms that comprise up to 1000 plies of uni-directional carbon prepreg tape and fabric near the blade root, where thickness is up to four inches, thinning out progressively to about a quarter of an inch at the tip. Plies are cut ultrasonically, the ply shapes having previously been downloaded from CATIA or AutoCAD. The preform is placed in a mould and resin is injected. Cure takes place in an autoclave or high-precision press. Resulting near net shape parts are milled to final dimensions on CNC machining centres. The composite parts are finally subjected to non-destructive inspection. To prevent blade damage from ingested matter, leading and trailing edges and tips are given a replaceable titanium cladding.
Carbon fibre blades are produced in a 160 000 ft2 (currently) composites facility at San Marcos, Texas, by CFAN, a 15-year- old joint venture between GE and French engine manufacturer Snecma. CFAN has an impressive track record in manufacturing highly contoured, thick laminate jet engine fan blades. More than 9000 of them are now in service, and production is expected to reach 3000 units per annum during 2007.
The carbon fibre blades have proved superbly reliable in service on GE90 engines, no special inspections or field actions having been required in over 7.5 million operating hours. Melvin Heard points out that despite over 100 bird strike incidents recorded, only three blades have had to be completely replaced. After such strikes, the titanium edges of blades can be ‘blended’ (made good by restoring their contours) while the engine remains on-wing, just as hollow titanium blades can. Maintainers may therefore continue to treat the new blades in the way they are used to with conventional blades.
GE has based its GEnx blades on those designed for the most recent mark of the GE90, the GE90-115B. Advances in design and materials have led to even fewer fan blades being used in the new engine, which has only 18. This reduces so-called scrubbing, the drag caused by air, disturbed by the passage of the previous blade, passing over a blade's surface. As a result, the engine runs more smoothly and quietly. Because of the lower blade mass, a lighter fan disc can be used to hold the blades in place. Teflon wear strips used where the blades dovetail to the disc minimise fretting and require no periodic lubrication or maintenance. As in the GE90, a titanium leading edge provides impact resistance.
CFAN is expanding its facility at San Marcos and gearing up to produce 1500-1800 GEnx blades per year by 2009.
Fan case
All-composite blades deserve an all-composite fan case, and that is what the GEnx designers have delivered – for the first time in a large turbofan engine. A weave of biaxial and triaxial carbon-epoxy braids has been specified, the biaxials being easier to lay in corners and bends. To fabricate the case, the braids are woven flat against a fan casing tool. A triple layer of triaxials laid mutually at 60° makes the weave thicker in the critical centre section of the sleeve than towards its ends. This all-composite construction avoids the need for the aramid (Kevlar)/aluminium containment system widely used in other engines, and the associated tendency for moisture to collect and cause corrosion at the interface between the two materials.
GE expects the GEnx case to be more resilient to ingested foreign object damage than previous designs, and to require less maintenance. Its concept is backed by some two decades of R&D including work carried out in connection with the Boeing Sonic Cruiser, an intended high-speed jet project that was eventually dropped in favour of the B787. Early ballistic impact panel tests have latterly been supplemented by ‘blade out’ testing using sub-scale blades at GE, Snecma and NASA's Glenn Research Centre. Tests have shown that an all-composite case can be more resilient than cases based on aluminium. A full rig ‘blade out’ test conducted at Peebles on a GEnx prototype last Spring proved successful, the case successfully containing all blade debris.
GKN Aerospace Services manufactures the fan containment case at its Aerostructures site in Tallassee, Alabama. A major expansion of the plant was initiated in 2005 to handle this addition to GKN's composite output. This sub-contractor is also producing an acoustic liner that substantially reduces fan noise. The single-piece liner comprises glassfibre/epoxy skins sandwiching a Nomex aramid honeycomb core.
Ducts
Another GEnx composite item outsourced by GE, in this case to EDO Fiber Innovations of Massachussetts, is a set of 10 ducts associated with the engine's variable bleed valves (VBV). Carbon composite was selected for its resistance to oxidation and because a complete duct set would weigh a mere 8 lb. Bismaleimide (BMI) resin is used so that the ducts can resist the effects of hot bleed air at high internal pressures.
EDO uses cost-reducing fibre placement and resin transfer moulding (RTM) techniques to fabricate the ducts from carbon fibre braid and BMI resin. Cytec Engineered Materials supplies the materials. During the braiding process, fibres are applied continuously to produce a preform, a method that avoids seams and consequential stress concentrations. Three layers of braid are used. The preform is placed in a mould, into which the resin is then injected. The RTM process ensures that both the inside and outside contours of the ducts are accurately defined, with a high-quality surface finish.
Promising
GE expects to certify the initial GEnx-1B64 mark of the engine, intended for the B787, in late 2007, ready for a mid-2008 service entry. GE's Mel Heard says that the engine will be highly efficient, burning 15.4% less fuel at cruise speed than the current-generation CF6. A later mark of the new engine, the GEnx-2B67, has been selected for the latest variant of the Boeing 747 jumbo, the B747-8 freighter, due to enter service a year later. Airbus may also use the GEnx for its planned riposte to the 787, the A350. Composites are key in enabling the engine to help meet airframer-set targets to drive 20% out of the cost of ownership for aircraft customers, by reducing fuel consumption and maintenance while enhancing longevity. Some 600 plus GEnx engines have been ordered so far.
Aviation faces stern challenges, not least growing environmental pressure to curtail its growth so that carbon emissions do not escalate. Composites can help square the circle, allowing some growth to continue without emissions rising too steeply overall. But the weight saving attributes of these adaptable materials must be fully exploited in engines as well as airframes if the full benefits are to be achieved. GEnx moves strongly in this direction and it looks as though this significant new engine, with its extensive composite content inside and out, has a promising future.