Composites are well-known for their exceptional mechanical and lightweight properties, but the feasibility of recycling these materials is limited. Most of them consist of a thermoset matrix, which cannot be recycled by melting or dissolution as in the case of thermoplastic matrices. Mechanical recycling is not a viable approach either, because the final result would be ground composite to be used as filler in new matrices at a great loss of value.
Another possible process – oxidation to recover the reinforcing fibers – may lead to a loss of fiber properties, along with complete resin degradation and the emission of greenhouse gases (GHGs).
Chemical recycling is therefore the only solution to the problem, as it can potentially be used to recover both reinforcing fibers and chemical blocks for use in the petrochemical industry or for synthesis of the same original resins. Two processes offer potential solutions: solvolysis uses chemical reagents to depolymerize the resin into its monomers or building blocks, whereas pyrolysis makes use of heat in the absence of oxygen to transform resin into a gas, liquid or solid residue. In both approaches, clean fibers can be recovered with very good retention of mechanical properties (up to 90%).
Recycling carbon-fiber-reinforced polymers (CFRPs) is of great interest now that the price of recycled fibers is comparable to the price of virgin fibers. Recycling can provide a representative reduction of the global warming potential (GWP) of 19-27kg CO2 eq. per kg CFRP and a reduction of primary energy demand (PED) of 395-520 MJ per kg CFRP. This is easy to understand from a comparison of the temperatures of the recycling process (400-600°C) and the manufacturing process (1.000-3.000°C).
Unfortunately, it is not true of recycling glass fibers, whose economic feasibility is one of the greatest challenges of implementing a large-scale glass fiber recycling process. This is also one of the main reasons for searching for other applications for glass fiber reinforced polymers (GFRPs) besides reuse as recycling technologies continue to develop and reduce operating costs.
Companies and countries, currently aware that the techno-economic difficulties of recycling end-of-life (EoL) composites, are putting the spotlight on ecodesign as a smart strategy to produce materials that are easier to recycle. This is the case of the ZEBRA Project (Zero wastE Blade ReseArch), which aims to manufacture 100%-recyclable wind turbine blade. However, until these initiatives are implemented, performance methods must be prepared for recycling future waste and to expand the number of applications for recovered materials.
To provide more context on the current problem, in the wind energy sector alone, 4,700 turbines are expected to be decommissioned in Europe from 2019 to 2023, which is equivalent to 40,000-60,000 tonnes. The International Air Transport Association (IATA) has estimated that 11,000 aircraft will be retired during the 2020s and that composites account for 10% to 50% of their total weight.
A success story: the EROS Project
In an effort to tackle the problem, Spain’s State Innovation Agency funded the 30-month EROS Project (an acronym derived from ’Economía ciRcular en compOSites’) to boost the circularity of composites by recycling waste from the wind energy and aeronautics sectors for repurposing in the ceramic and transport industries. It started in July 2020 and involved two technology centers (AIMPLAS and ITC), two companies in the ceramic industry (KERABEN and FRITTA), one recycling company (RECICLALIA) and one company in the transport industry (SOFITEC).
EROS started by collecting waste from decommissioned wind turbine blades and parts from end-of-life aircraft, which was chopped and shredded to a particle size of 5mm. A crucial separation step was required for the ground waste from turbine blades because they usually have external layers of polyester resin reinforced with glass fibers, a wood or PVC core along with adhesives, gel coats and some metal parts.
This separation was carried out using air elutriation in a zigzag pattern at AIMPLAS’ facilities and a pure fraction of a glass fiber reinforced polymer was obtained (see d) and e) in Figure 1). The recycling of both kinds of waste was assessed using pyrolysis and solvolysis.

Figure 1: a) Pieces of cut wind blades; b) Pieces of cut GFRP from aircraft; c) mill for grinding composites; d) AIMPLAS elutriation separation system; e) light fraction of GFRP obtained after elutriation; f) light fraction of CFRP obtained after elutriation. (images: AIMPLAS)
Pyrolysis proved to be an efficient approach for recycling both kinds of waste and depolymerization yields of more than 90% were achieved for a process lasting less than two hours. Furthermore, the glass fibers were recovered with a carbon content of less than 0.1% by mass, thus satisfying the ceramic industry’s requirements for reuse in their demo samples.
The process was carried out in two steps. The main one was pyrolysis at temperatures in the range of 450-550°C, from which fibers were obtained with a deposition of char. In the next step, this char was eliminated by oxidation at a carefully controlled process temperature to obtain clean fibers without damaging their surface (see Figure 2).
In the case of the glass fiber, it is important to remove this char so that no residual organic matter interferes with manufacture of the ceramic demo samples. For carbon fiber, a clean surface is crucial for subsequent application of the sizing agent. Because pyrolysis reactors have a higher capacity than solvolysis reactors, thermal cracking was selected to scale-up the recycling process at a kg scale, which was the required scale to make the demonstrators and measure their properties.

Research was also done into solvolysis recycling with the twofold goal of obtaining clean recycled fibers and glycols for reuse as solvents in ink formulation in the ceramic sector. A wide variety of subcritical (atmospheric pressure and low temperature) and supercritical (high pressure and temperature) conditions were tested, using different types of catalysts (metallic salts, inorganic bases, organic acids) and solvents (water, alcohols, amines), and depolymerization yields of 85%-95% were obtained.
The process temperature was significantly lower (250-325°C) than for pyrolysis, but the reaction times were longer (6-16 hours, compared to 1 hour on average for pyrolysis), after which the fibers were filtered and cleaned (see Figure 3). This technology may therefore be of interest to maintain the fibers’ mechanical properties and reuse the depolymerization products in applications such as ink formulation and even to synthesize new polymers.

The glass fibers were then subjected to elemental analysis to determine their carbon, fluorine, chlorine and sulfur content. The presence of carbon is related to black imperfections on ceramic media while the presence of the other elements is responsible for emission of acid compounds when ceramic tiles are baked. As with pyrolysis, the total carbon content of the samples was less than 0.1%.
Recycling potential
Elemental analysis showed that the glass fibers had a composition that was very suitable for the required application, with a boron oxide (B2O3) content of approximately 6% in mass. This oxide is highly appreciated for its ability to lower the glass melting temperature and is characteristic of E-glass – the most common kind of glass fibers used to manufacture wind blades – which confirms the suitability of this waste for new applications.
These clean fibers could not be used directly after the recycling process, however, because they were very fluffy, which made processing difficult. They were therefore milled by ITC in a hammer mill to adapt their grain size to tile production. During handling, the freshly-milled fibers had a tendency to agglomerate, although this was beneficial for subsequent processing (Figure 4).
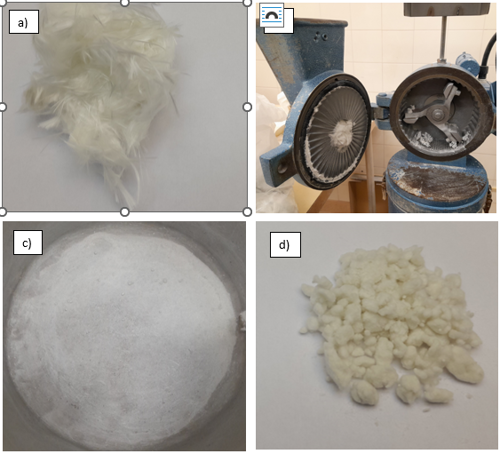
Two ceramic applications were tested: ceramic media and ceramic frits. In the case of frits, FRITTA worked on the recovered glass fibers by making opaque and transparent frits using a modified frit formula that incorporated 3% and 6% recycled glass fiber (rGF) to the mixture. The resulting frits were then mixed with water, kaolin and additives ground together to obtain a suspension that was applied to ceramic tiles and baked to manufacture the samples.
These frits showed almost no deviation in quality control parameters, such as brightness at 60°, dilatation rate and temperature behavior, thus confirming the suitability of rGF for use in this kind of product, and suggesting that even more rGF could be added to frits (Figure 6).
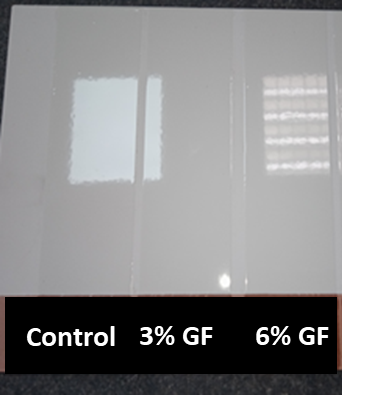
In the case of ceramic media, ITC studied whether glass fibers could replace feldspars and the effects on the samples’ rheological behavior in terms of apparent density and gresification curves, as well as final post-baking properties such as porosity, mechanical properties and density.
It was concluded that 10%, in mass, of glass fibers could be used without affecting the final properties, although a slight reduction in the ceramic media baking temperature was observed, which is positive in terms of energy reduction. The media with and without fibers did not present macroscopic differences, thus opening the door to including this waste without affecting the final quality of the material (Figure 5).
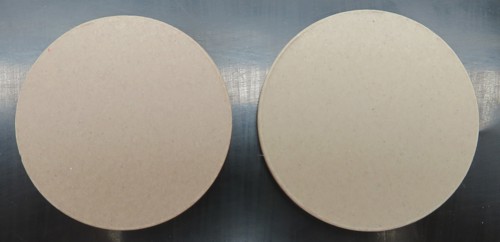
Processing
The recovered glass fibers could be directly used to manufacture ceramic media, but the carbon fibers had to be coated with a sizing agent to enhance their compatibility with the epoxy resin in the final demo sample.
For this purpose, AIMPLAS studied the effects of different commercial (chemicals sold by suppliers for that purpose) and non-commercial (chemical reagents with potential use as coatings) sizing agents, their concentration, the amount of solvent and number of layers of coating applied to the fibers. Moreover, a new method for applying the sizing agent was tested and showed the best results with non-commercial sizing agents. Water consumption was also reduced by more than 100%.
The coating’s effect was analyzed and showed an improvement of 18-41% in interlaminar shear strength, 45-75% in tensile strength and 80-86% in compressive strength when the selected non-commercial sizing agent was compared with two different commercial sizing agents. The coated fibers are being processed by the aeronautics partner (SOFITEC) in charge of manufacturing the final demo samples using sheet molding compound (SMC), resin transfer molding (RTM) and infusion.
Future projects and new applications
AIMPLAS is currently searching for other applications for recovered fibers, such as their use in asphalt mixtures and concretes to reduce those mixtures’ material fatigue and weight and to provide them with better mechanical properties and strength for the environment compared with existing materials.
Considerable work in research, development and application of sizing must be done to adapt the fibers for use in matrices that have not been explored in the project while taking into account crucial factors such as hydrophilicity, corrosion resistance, length and optimal percentage of recycled fibers. AIMPLAS is also doing research into reusing depolymerization products, in particular pyrolytic oil and solvolysis mixtures, which is key to increasing the economic feasibility of the process and helping improve the framework by including the petrochemical industry and resin manufacturers.
1. Meng, Fanran; Pickering, Stephen & Mckechnie, Jon. (2018). An Environmental Comparison of Carbon Fiber Composite Waste End-of-life Options.
2. WindEurope (2019) Market outlook to 2023.
3. The International Air Transport Association (IATA). Helping Aircraft Decommissioning. 2020. Available online: https://www.iata.org/en/programs/environment/aircraft-decommissioning/ (accessed on 17 December 2020).
4. https://www.compositesworld.com/articles/fabrication-methods