

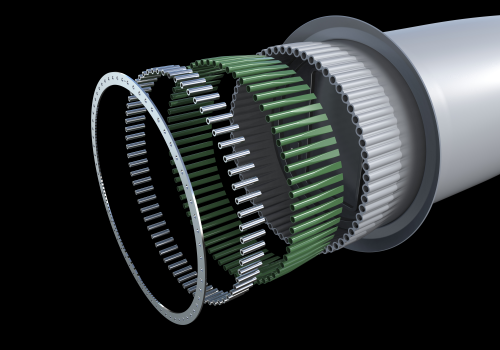
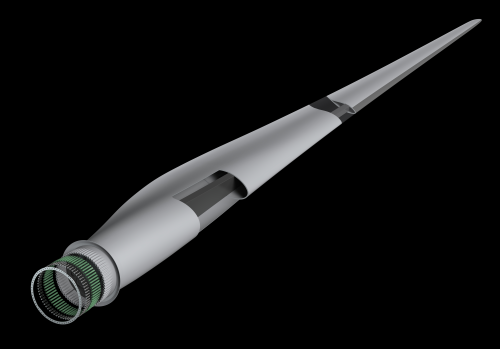
A key concept behind this bold assertion is that, instead of developing ever larger monolithic fabrications, a better option is to go smaller.
We believe that the present approach to up-sizing, as you get towards the 6-10 MW machines now in prospect, will bring horrendous issues of repeatable quality and that the cost of overcoming these will be prohibitive. |
Paul Rudling, chairman, Blade Dynamics |
Explaining this paradox, company founder and chairman Paul Rudling says: “We’re turning conventional wisdom on its head. Instead of making larger components to produce bigger wind turbine blades, we’re scaling down. We believe that the present approach to up-sizing, as you get towards the 6-10 MW machines now in prospect, will bring horrendous issues of repeatable quality and that the cost of overcoming these will be prohibitive. There comes a point at which it makes no sense to produce blades in ever bigger units. Thicker composite sections are hard to cure and prone to voids, mould tools become vast and the logistics become impossible. Bonding together outsize parts, such as the shells that form the blade envelope, is fraught with difficulty; witness, for instance, the fact that wind energy adhesives often have to pass a one-inch slump test!”
“Therefore,” Rudling expounds, “we’ve had a fundamental re-think. Instead of taking a conventional approach to blade production, why not make a number of blade components of manageable size, using well-proven technologies, sensible tooling, established quality systems and average factory floor space? You could produce high-quality modules in normal factories and transport them by normal means to a final assembly area close to where the wind turbines will be deployed. There you’d bond them together into the complete blade.”
This is counter-intuitive to the prevailing mind set which considers that, to have integrity, a structure has to be monolithic.
“But that is just not so,” Rudling declares. “Formula 1 cars, high-tech race boats and aircraft are made by bonding together dozens if not hundreds of parts. The technology for producing good joints is well understood and, if they are carefully made under controlled conditions using quality materials, there is little risk. We can build quality into our joints far more reliably than can the builders of large monolithic structures, by applying well proven bonding and testing processes that are not out of their depth in terms of scale.”
Though this ‘scaling down to scale up’ philosophy is the most attention-grabbing aspect of Blade Dynamics’ approach, it is far from being the whole story. It is just one part of a fundamental re-examination of design and manufacturing processes aimed at making blades longer. Conventional technology, says Rudling, is running into the buffers presented by the empirical rule that energy capture increases with the square of rotor radius whereas blade mass increases by a cube factor.
“If you could make rotors larger for the same weight and inertia, that would extend present limits, increasing the amount of energy that can be harvested.”
According to Blade Dynamics’ sales director Theo Botha, current composite fabrication is flawed because much of it is rooted in decades-old technology first used in mass market boat building. Such methods are, he argues, relatively crude and not suited for large high-performance structures such as wind turbine blades. Nor are they particularly effective with the carbon composites that are increasingly used in the largest blades for their properties of stiffness and low weight. As a result, quality is highly variable. The measures designers must apply to allow for this variability result in structures that are bulky and overweight. By going back to basics, Blade Dynamics is providing a slimming treatment. Blade design and manufacturing processes are equally under review. The overall aim, says Botha, is to make wind energy more competitive with other energy sources.
A clean sheet approach
Despite being only five years old, Blade Dynamics is no brash upstart and has well rooted antecedents. Chairman Paul Rudling is a seasoned composite and corporate professional, having previously founded SP Systems and nurtured it, over three decades, to become a dominant innovator of advanced composite technology in high-performance structures. After SP Systems was acquired by Swiss composites house Gurit AG, Rudling went into semi-retirement for a short period then decided to tackle the deficiencies he thought were arising in wind turbine blades, especially in the larger blade sizes. A new company, he reasoned, would be able to take a clean sheet approach, free from the shadow of legacy technology that more established players must contend with.
To hasten its evolution, the fledgling company teamed up with partners American Superconductor Corporation (AMSC) and the Dow Chemical Corporation (see Dow Venture Capital invests in Blade Dynamics Ltd.) This brought in additional finance as well as a fruitful partnership with the wind energy interests of AMSC and backward integration into the composites supply chain through Dow. Meanwhile, Rudling had been building an expert team that includes “some of the best brains in composites and wind energy.” Their brief was to take nothing for granted and to re-examine every aspect of blade design and manufacture. By considering afresh everything from basic configuration to the specifics of roots, spars, skins etc, they are expected to deliver a ‘game changing’ wind turbine technology that could increase AEP (annual energy production) by up to 10%, with commensurate reliability and durability improvements.
Partnering with American corporations has also facilitated access to a substantial manufacturing site in Michoud, near New Orleans, Louisiana. A 100,000 ft factory on a 43-acre site leased from NASA, which once used it to produce propellant tanks for the space shuttle, has extensive quayside access to the Mississippi so that blades, jigs and tools can be transported by river and sea, free from the size and form limitations of road and rail travel.
Explaining the relationship between the UK and US establishments, Rudling says: “The Isle of Wight is our ‘skunk works’ [a reference to the iconic R&D centre responsible for many ground-breaking innovations for the Lockheed Martin Corporation - Ed], whereas Michoud is our centre for advanced manufacturing, especially for the American market. We may also in the future manufacture in the UK for the European market. These are the two markets we are targeting initially.”
First blade
The blade is made up of a number of major sub-components that are subsequently bonded together. All the components are transportable in 40 ft containers. |
While the company has found a useful revenue stream in consultancy, its first tangible product is the Dynamic 49 blade, the first of which has been manufactured at Michoud and is now undergoing extensive evaluation. The company claims that this new product, with its several patented technologies, redefines blade design and manufacture to provide a step improvement in performance versus cost. In line with the philosophy outlined above, the blade is made up of a number of major sub-components that are subsequently bonded together. All the components are transportable in 40 ft containers. Carbon/epoxy composite is used for the hollow main spar and other skeletal elements where stiffness and strength are key, while the envelope comprises low-weight glass reinforced plastic (GRP)/foam sandwich skins. Novel techniques are used to assemble the carbon spar, and it has not been necessary to incorporate pre-bend.
At this stage, the company is sparing with details. It claims that, in general, it is not wedded to any particular materials or processes, explaining that these will vary according to the component, manufacturing location and the required combination of performance, quality, manufacturability and affordability. Clearly it will seek material combinations that provide strength, stiffness and low weight together with high fatigue endurance and durability. It will, for example, have taken note of recent advances made by its partner Dow in resins and foams.
Significantly, Dow resin technologists claim to have broken the link between low peak exotherm – desirable because of the damage that excessive thermal excursions during cure can do to the laminate – and low reactivity, or speed of cure. It says that its new AIRSTONE epoxy resin system reduces exotherm while maintaining cycle times. Moreover, the resin’s low viscosity makes for faster and more complete infusion through multi-layer structures. Dow also offers new Germanischer Lloyd (GL) approved structural foams said to improve on existing polyvinyl chloride (PVC) and polyethylene terephthalate (PET) materials in terms of their weight, mechanical properties and high cycle fatigue tolerance. COMPAXX foams also absorb little resin during blade manufacture, so that weight is not added, and bond well with skin composites (see Dow introduces COMPAXX wind blade core material.)
Blade Dynamics ran hundreds of specimen tests before alighting on the material and process combinations it has selected for the Dynamic 49. As a result of its careful attention to detail, it expects the 49 m long blade, intended for 2 MW turbines such as that produced by AMSC, to weigh some 5900 kg, compared with about 8 tonnes for some competing rotors of similar size. It can therefore provide a larger rotor for existing 2 MW machines without over-loading bearing mechanisms, shafts and other drive train components. Typically, a 93 m rotor becomes 100 m.
Carrots and skins
While most of the innovations emerging from the skunk works are still under wraps, two are now sufficiently protected to be cited as examples. These involve the critical root attachment area and blade surface protection.
A fundamental re-think of the way the blade-hub attachment is normally engineered convinced the design team that present arrangements are archaic, heavy and inherently unreliable.
“Installing large constant-diameter threaded bolts into thick composite sections by drilling and bonding did not seem the way to go,” explains Rudling.
“Loads exerted on the bolts get concentrated onto bolt ends and adjacent threaded portions, so that load bearing and transfer are uneven. Added to which, load is transmitted from the bolt into the laminate via an interface layer which, inevitably, is resin rich and fibre deficient so that is another weakness. It’s like trying to secure something by driving a screw into end-grain wood.”
Engineers have had to compensate for these weaknesses by over-specifying the whole joint – laminate, bolt and all – if joints are to bear, over a 20-year life, high gust loads and resist long-term fatigue effects. This results in excessive weight, inertia and material usage. Blade Dynamics engineers have addressed this attachment difficulty with a composite root insert. This looks a bit like a carrot, the name often given in the industry to the metallic insert normally used for the purpose. The new ‘carrot’ insert tapers from a bulbous cylindrical outboard end to a thin wedge inner, this shape being designed to avoid thick edges and hence stress concentrations so that load can instead be transmitted progressively into the rotor hub laminate.
The new carrot root insert comprises a composite outer jacket which envelopes a threaded metal socket that will receive the blade attachment bolt. The metal socket is spiral-threaded on the outside as well as appropriately threaded on the inside to receive the specified securing bolt. The unit is manufactured in such a way that resin-impregnated composite fibres are wrapped round the spiral-threaded socket, ensuring a metal-to-composite interface having properties that are fibre dominated rather than resin dominated. Each fibre helps transmit load from the attachment bolt through the socket and into the laminate; this should ensure that the joint is both strong and durable.
The complete root insert can be coated with adhesive and bonded into the hub laminate, or inserted as part of a secondary infusion process. This results in a firm bonded fit which, thanks to the large bonding surface area afforded by the bulbous end and the clever distribution of fibres, will ensure a highly secure composite-to-composite joint once the resin has cured.
Thanks to the higher effectiveness and security it confers, the novel root insert enables designers to engineer hub and root structures that are slimmer and lighter than they would conventionally need to be. Benefits from this accrue throughout the drive train since a lighter root and hub enable designers also to specify lighter drive shafts, bearings and associated components. Under test, the Dynamic 49 root insert has proved able to withstand loadings above 200 kN, well in excess of extreme gust requirements, and offers vastly improved fatigue performance over more conventional designs.
The second revealed improvement is a blade finishing process patented under the name BladeSkyn®. This involves the application of a thin (130 micron) surface film derived from aerospace practice and based on thermoplastic nanotechnology. The material adheres well to lightly sanded glass/epoxy composite and has complete opacity in the range of colours used in the wind turbine market. By protecting against pitting and abrasion, it prevents the progressive degradation of aerodynamic performance that affects blades having standard gel-coat and paint finishes. It resists rain, ice, UV, chemicals and solvents; can endure service temperatures of -40°C to +80°C, has very low reflectivity and repels dirt. Coating blades in this way means that surface properties are pre-assured, with much lower variation than with conventional finishes. It also avoids the need for flatting back and other finishing operations that can degrade surface properties.
BladeSkyn’s additional cost is said to be modest compared with the O&M (operations and maintenance) savings that it secures through life.
Other innovations and new products can be expected as Blade Dynamics continues with its drive to re-think and update wind turbine blade design and manufacture.
As Paul Rudling summarises: “It’s the kilowatt hours a wind turbine produces that matters, not its nameplate rating. Increasing the area swept by the rotor for the same weight and inertia can increase kilowatt hours by 10%. That’s a prize worth having and that is what we are in the process of delivering.”
Breaking news
In January, the Energy Technologies Institute (ETI), a public-private partnership between industries and the UK government, announced the appointed of Blade Dynamics to develop and demonstrate technologies for constructing blades of 80-100 m in length, incorporating carbon fibre.
The blades are intended for use on the next generation of large 8-10 MW capacity offshore wind turbines currently under development. Currently 5-6 MW capacity turbines are deployed offshore, featuring blades of 60-75 m in length.
According to the ETI, the blades will weigh up to 40% less than conventional glass fibre based blades, enabling significant weight and cost savings to be achieved throughout the rest of the turbine system. As part of the £15.5 million project, the ETI will become an equity investor in the Isle of Wight-based blade developer, helping with technology development and allowing the company to grow its workforce by up to a third in the short to medium term.
The project will involve the manufacture of prototype blades, ready to be put into production by late 2014. Structural testing for the first blade is then expected to be carried out at a UK test facility.
The first stage of the project will focus on blade design in collaboration with a major turbine manufacturer (OEM). The project will also test detailed design and manufacturing technologies, extending Blade Dynamics’ current experience from manufacturing 49 m blades. The second stage will establish and demonstrate the proposed manufacturing processes on blades designed for a current 6 MW turbine. A design will also be developed for blades for future 8-10 MW turbines. Final project stages are intended to test and verify the prototype blade performance against the predicted performance.
“Offshore wind has the potential to be a much larger contributor to the UK energy system if today’s costs can be significantly reduced,” notes Paul Trinick, Offshore Wind Project Manager at the ETI.
“Investing in this project to develop larger, more efficient blades is a key step for the whole industry in paving the way for more efficient turbines, which will in turn help bring the costs of generating electricity down. Along with improved system reliability, the impact of larger blades is a crucial factor in helping to bring down the costs of generating electricity offshore. Our investment strategy here is to provide financial support to allow the company to develop its technology further, to accelerate and expand the testing of this UK technology, and to identify the large-scale development opportunity of this design approach.”
“Financial backing from the ETI for this project allows deployment on ultra-large turbines far sooner than would otherwise have been possible and as a result of this project we will be hiring new engineers and technologists to make this possible,” adds David Cripps, Senior Technical Manager at Blade Dynamics.
“Our driver is to make the generation of electricity through offshore wind both more reliable and more economical. We believe longer, low weight blades to be a key part of the solution, but for such blades to be most effective we need to design their construction differently.” ♦
Also see:
New materials tackle weight, strength and cure time issues for wind turbine blades Bernd Hoevel, Senior R&D Manager, and Alain Sagnard, Senior R&D Engineer, at the Dow Chemical Company, describe how the company's foam core material and epoxy resin systems can help manufacture lighter, stronger wind turbine blades.
This article was also published in the January/February 2013 issue of Reinforced Plastics magazine.
The digital edition of Reinforced Plastics is distributed free of charge to readers who meet our qualifying criteria. You can apply to receive your free copy by completing the registration form.